Moisture Content and Water Activity
Water or moisture content in food does not determine food safety or predict product shelf life as the water in food exists in three states, bound, capillary and free water, each with a different availability to support microbial growth and deteriorative chemical reactions. Foods with the same amounts of water may perish at very different rates; thus, water activity (aw), or the amount of water in equilibrium available to hydrate food components and a measure of the energy status of water in the system more accurately predicts deteriorative reactions and derived from laws of thermodynamics. Water activity is the ratio of the fugacity (f) of water in food compared (fo) to the fugacity of pure water, and fugacity represents the tendency of water to escape from the system and closely relates to vapor pressure; hence water activity may be rewritten as:
aw=(f/fo)~(p/pi*)
where is the vapor pressure established above the food and pi* is the vapor pressure of pure water at the same temperature. Adding solutes and macromolecules to water decreases the entropy of the solution relative to pure water, resulting in a decreased vapor pressure, a colligative property. Materials have an aw between 1 and 0 (100% and 0% relative humidity) for pure and no water systems, respectively. Water activity, aw, correlates with microbial growth, deleterious reactions, oxidations, and vitamin and color degradation.

Bound, Capillary, and Free Water

Bound water cannot be removed at 100 oC as it forms strong non-covalent interactions with other food components. Bound water interactions are strong non-covalent ionic, typically found with salts and proteins with ionizable amino acids, hydrogen bonding with sugars and amino acids as monomers and polymers, and dipole–hydrogen bonds with polar molecules and polymers. The limit of bound water, to a maximum aw of 0.5, is described as the Brunauer–Emmett–Teller (BET) (Brunauer, 1938) monolayer, which originally described the physical adsorption of gas molecules on a solid surface; but adapted in Food Science to describe the physical absorption of water onto surfaces.
Moisture sorption isotherms describe the relationship between water activity (aw) and moisture content (Mw), and the BET model is commonly used to characterize the relationship between moisture content and water activity and to calculate the limit of monolayer-bound water (Mo) in dehydrated foods where C is a constant.
Salts, sugars, and other soluble components added to water form strong non-covalent interactions (i.e., dipole-dipole, ionic and hydrogen bonds), and water becomes unavailable to support chemical reactions or microbial growth and is termed bound water. In more complex systems, such as bread, meat, and pasta, water can be confined in narrow gaps between surfaces and is held in place by surface tension and termed-capillary water. Capillary water affects the vapor pressure differently than bound water as it is more available to support deteriorative reactions and more readily escapes into the vapor phase than bound water.
Free water is not closely associated with surfaces, nor does it forms strong interactions with other food molecules, and is readily removed by drying. Free water in foods interacts via hydrogen bonding with other water molecules, similar to bulk water and is available to support microbial growth and chemical reactions. Salts, sugars, and other soluble components added to water form strong non-covalent interactions (i.e., dipole-dipole, ionic and hydrogen bonds), and water becomes unavailable to support chemical reactions or microbial growth and is termed bound water. In more complex systems, such as bread, meat, and pasta, water can be confined in narrow gaps between surfaces and is held in place by surface tension and termed-capillary water. Capillary water affects the vapor pressure differently than bound water as it is more available to support deteriorative reactions and more readily escapes into the vapor phase than bound water. Free water is not closely associated with surfaces, nor does it forms strong interactions with other food molecules, and is readily removed by drying. Free water in foods interacts via hydrogen bonding with other water molecules, similar to bulk water and is available to support microbial growth and chemical reactions.

Water Activity, Food Quality, & Stability
Water activity affects food quality and stability, including microbial spoilage and enzymatic and non-enzymatic deteriorative reactions. The aw of food is reduced by adding humectants (bind water) or by removing water during drying. Water in foods at, or below, 15% is bound in the BET monolayer and corresponds to low aw, as the water is closely associated with monolayers and multilayers and does not participate in hydrating microbes nor partaking in the mobility of enzymes. Intermediate moisture foods (IMF), with 20 to 40% moisture, exceed monolayer and multilayer water; water is somewhat confined as capillary water. IMFs are highly susceptible to enzymatic reactions and, to a lesser extent, microbial spoilage. High moisture foods, > 40% moisture, undergo rapid spoilage if no alternative processes have been applied to limit deleterious reactions from occurring. aw predicts the occurrence of different types of food spoilage; for example, at an aw less than 0.65, no microbial spoilage occurs, which exponentially declines with decreasing aw. Gram-negative bacteria are adapted to low osmolarity and found in highly dilute foods, unable to grow in foods with a water activity less than 0.97; while gram-positive bacteria can survive and proliferate at water activities as low as 0.9. The outer lipopolysaccharide for Gram-negative bacteria restricts water and nutrients from transporting into the cells making their water requirement greater than Gram-positive bacteria. Yeast and molds survive in lower moisture foods than bacteria and tolerate lower water activity 0.7. Not all correlations between water activity and spoilage rates are as simple as that observed for microbial growth. For example, lipid oxidation occurs at the slowest rate when the aw is near 0.2 and is attributed to small amounts of water required to bind hydroperoxides; and more water added to the system aids in solubilizing oxygen into the food matrix, increasing the rate of oxidation to a maximum at an aw of 0.75, after which the high water activities dilute substrates effectively slowing oxidation. The Maillard reaction exhibits maximum activity at an aw of 0.75 due to feedback inhibition when water is an end product.
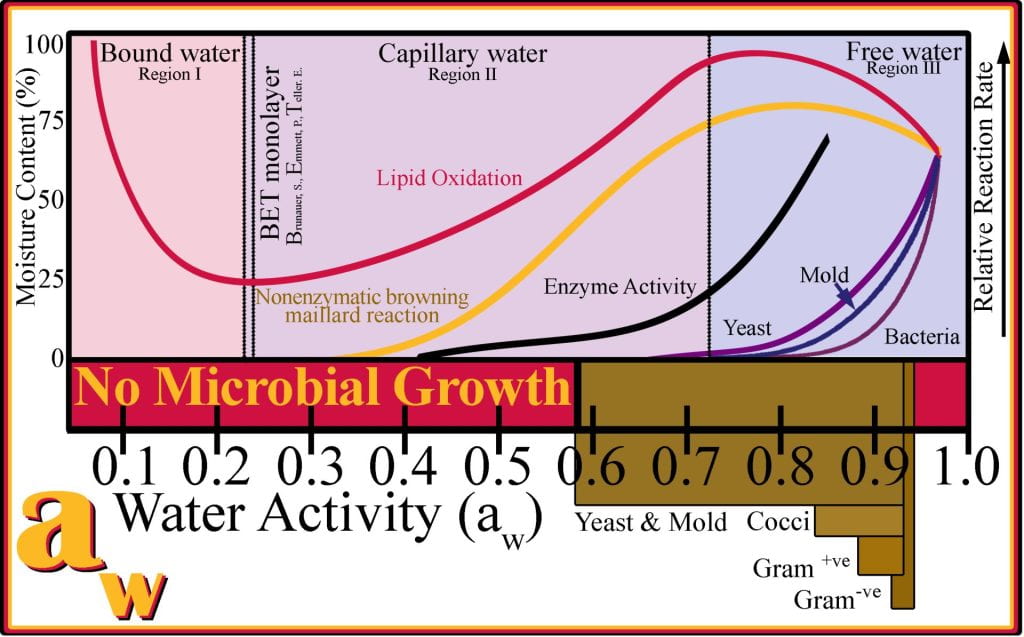
Works Cited
Brunauer, S., Emmett, P. H., Teller, E. 1938. Journal of the American Chemical Society. 60: 309