Lipid Modifications
Hydrogenation
Fats and oils impart physical structure to a wide range of whole food (e.g., milk products, meat, and fruits such as olive and avocados) and ultra-processed formulated foods (e.g., plant-based meats, pizza, frozen dinners, cookies, cake, ice cream, chocolate, and pastries), most of which require solid fat (long-chain saturated fatty acids) to impart the desired sensory and organoleptic properties. Oils are liquid at room temperature with high concentrations of unsaturated (oilseeds, corn, olive) or medium-chain (coconut, palm kernel (fractionated)) fatty acids. Oil seed crops (soybean, sunflower, canola, flax, peanut, cotton) are 20-40% lipid with high concentrations of mono or polyunsaturated fatty acids, making them liquid and devoid of fat crystals. Foods formulated with refined oil include sauces, salad dressing, mayonnaise, and plant-based meats or added during frying (e.g., French fries, donuts, and potato chips). Solid fats are high in long-chain saturated fatty acids, which form solid colloidal fat crystal networks at room temperature and impart essential physical structure and organoleptic properties. Corn, peanut and Oilseeds are grown across Canada (edible oil exporter); however, domestic solid fat production (e.g., milk fat, lard (pork fat), and to a lesser extent, eggs) requires imports of solid fat, as the climate prohibits the cultivation of Elaeis guineensis (oil palm tree) and Theobroma cacao (cocoa bean). All commodities, high in saturated, solid fat, face significant challenges; animal welfare and environmental sources place pressure to move away from conventional animal sources, coupled with governments limiting the export of palm oil and cocoa beans to curb deforestation, leading to the need to create solid fat.
Hydrogenation adds molecular hydrogen to each carbon adjacent to a fatty acid unsaturated bond using a heated, pressurized hydrogen gas vesicle where the oil and catalyst, usually nickel but also palladium or platinum, blend is mixed, allowing hydrogen gas to pass through the slurry.
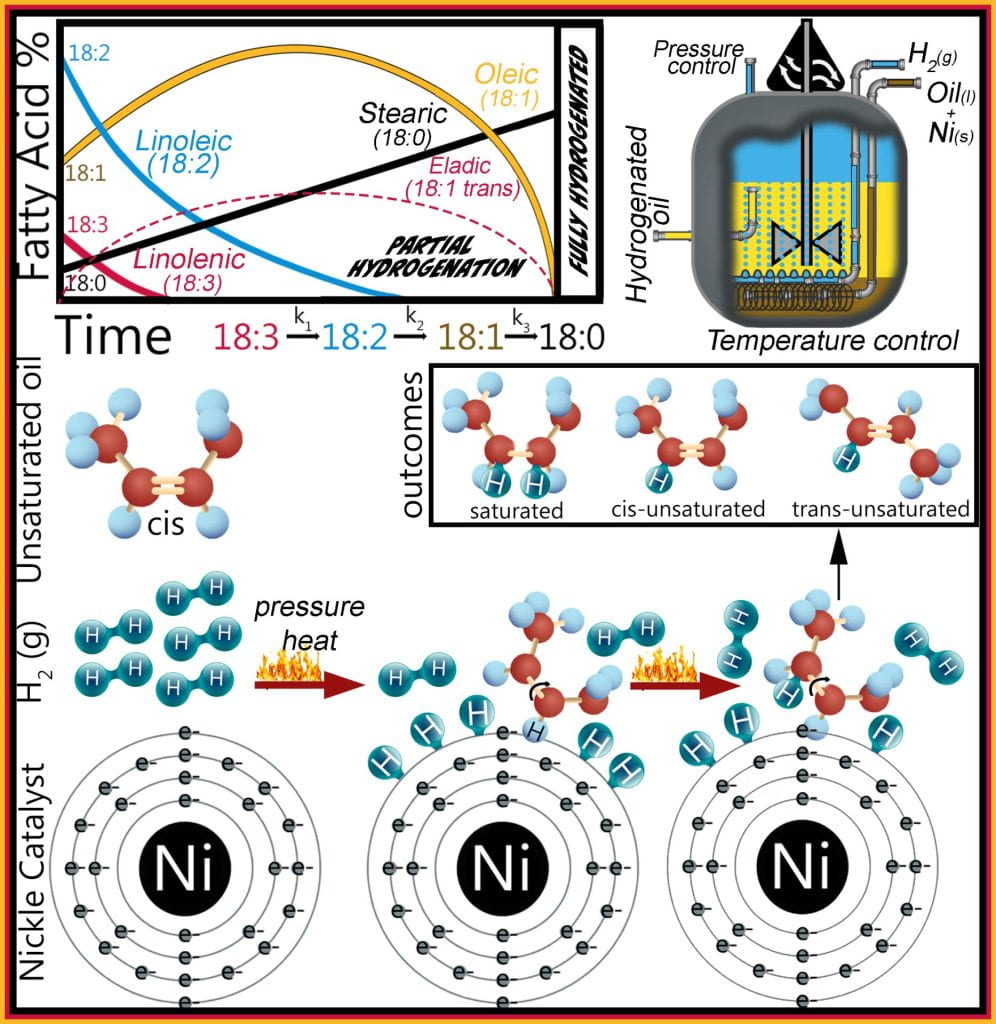
The Horiuti–Polanyi mechanism sequentially describes hydrogenation, where molecular hydrogen is adsorbed onto the nickel surface (step 1) and dissociates into two hydrogen atoms (step 2). Concurrently, fatty acids are also adsorbed onto this nickel surface by their double bond, which forms a half-hydrogenated intermediate (step 3). If a second hydrogen atom (step 4) is added to this intermediate, the original double bond is saturated and no longer reactive; but since the first hydrogen addition (step 2) is reversible, the intermediate can also dissociate from the catalyst and reform an unsaturated bond. This process repeats for each point of unsaturation; thus, linolenic (18:3) transforms to linoleic (18:2), then to oleic (18:1) and ends with stearic (18:0) acid formation.
The half-hydrogenated intermediate is reversible, allowing the unsaturated double bond to reform, which either adopts the cis or trans configuration and is the primary source of industrially produced trans eladic acid. Originally methods to attain the desired viscoelastic properties of the fat employed partial hydrogenation of the liquid oil, reducing the unsaturated fatty acids content while increasing both the saturated and trans unsaturated fatty acid concentrations transforming the liquid oil into solid fat. However, recent legislation prohibits foods containing industrially-produced trans fats banning partial hydrogenation. Hydrogenation is the only method to increase the solid fat content of liquid oils, transforming them into plastic fats with desirable functional and sensory properties. Oils are still hydrogenated; however, the reaction runs to completion to ensure that the liquid oil is fully hydrogenated, ensuring only saturated fatty acids remain while preventing trans fats from forming. The downfall to fully hydrogenated fats is their extremely high melting temperatures giving them a plastic organoleptic perception with limited functional properties.
Blending and Interesterification of Lipids
Fully hydrogenated fats are not directly added to foods because they have poor physical properties such as high melting points (mp>50oC) and hard waxy structure, which are imparted by the fully-saturated, long-chain TAGs. When fully hydrogenated fats are blended with liquid oil (e.g., corn, oilseed, olive) and melted above their melting temperatures to form a solution, upon cooling, the two lipid sources do not co-crystallize, and the product quickly phase separates (crystals precipitate out of oil-crystal dispersion) as graining or coarsening occurs (Ostwald’s Ripening).

Liquid oils blended with fully-hydrogenated fats are made functional by the chemical processes of inter- and intraesterification. Interesterification removes and then exchanges fatty acids between TAGs, while intraesterification exchanges fatty acid position on a single TAG. Interesterification transforms an unfunctional blend of corn oil, high in monounsaturated TAGs, and fully hydrogenated corn oil, high in tristearin (glycerol with three stearic acid fatty acids) into a functional blend by randomizing fatty acid position between TAGs. Interesterification does not change the fatty acid composition; it only modifies their TAG position. The resultant interesterified fat now has a broad distribution of TAGs resulting in a broad melting profile. Blends of oils and careful control of the interesterification conditions are used to tailor the sensory and physical properties mimicking functional fats such as cocoa butter and milk fat. Numerous catalytic methods include enzymatic and chemical synthesis, which randomizes fatty acids differently, coupled with careful temperature control, directing the blend toward a composition with the desired melting profile.
Bromination of Lipids
Emulsion destabilization arises due to divergent densities between the continuous and dispersed phases leading to the creaming of the lower-density phase. Brominated vegetable oil (BVO), typically around 8 ppm, has been employed since early 1930 because droplets containing BVO remain suspended in the water. Bromination lipids are applied to beverages containing citrus oil to prevent separation before expiry. Unsaturated oil is brominated to diminish the density differences between the continuous aqueous phase from the lipid-dispersed phase. Adding bromine to unsaturation points along the fatty acid chains increases lipid density; for example, lemon oil has a density of 0.88 g/cm3, while after bromination, the density increase to 1.33 g/cm3. The density of the continuous water phase is matched by blending three parts lemon oil with and one part brominated lemon oil. Eliminating the density difference between particles eliminates the buoyant force acting on the particle, imparting stability.
BVO had its GRAS (generally regarded as safe) status revoked in 1970 and was replaced with food additive regulations, restricted to 15 ppm in the United States. The only allowable use of BVO in Canada currently in beverages containing citrus oils; BVO is completely banned across the European Union.

Starch Modifications
Starches are modified with chemical, thermal and nonthermal or physical processes. Starches are modified to different extents where the degree of substitution (DS) for modified starch is targeted within high (1.5–3), medium (0.2–1.5), and low (0.01–0.2). The DS alters their applications as thickeners and stabilizers. Starch hydrolysis decreases their viscosity when in solution; however, the shorter dextrins produced become increasingly soluble and provide body and texture to confectionary products. Starches are modified with chemical, thermal and nonthermal or physical processes. Starches are modified to different extents where the degree of substitution (DS) for modified starch is targeted within high (1.5–3), medium (0.2–1.5), and low (0.01–0.2). The DS alterstheir applications as thickeners and stabilizers. Starch hydrolysis decreases their viscosity when in solution; however, the shorter dextrins produced become increasingly soluble and provide body and texture to confectionary products.
Etherification, Esterification of Starch

Etherification of starch is mainly done to inhibit retrogradation, typically using propylene oxide, which adds a hydroxypropyl group onto starch. The introduction of a hydroxypropyl group alters polymer alignment leading to opaque gels. Esterification of starch modifies starch via acetylation, phosphorylation and succinylation. Monostarch phosphate (E1410) is added to products to prevent starch retrogradation. Phosphate distarch phosphate (E1413) is a chemically modified starch that replaces interchain hydrogen bonds with stronger permanent, covalent bonds between phosphates by treating starch with phosphoric chloride (POCl3). E1413 thickens soups, sauces and gravies and limits ice crystal growth during the freeze-thaw cycle.
Succinylation & Cationic Starch
Succinylation of starches increases resistance to digestion while the succinyl group weakens the inter-molecular bonding facilitating swelling, solubilization and lower temperature gelatinization. Cationic starch varies in substitution from a low degree (< 0.07) to a high degree of substitution (≥0.07); a low substitution starch is positively charged and attracted to the negatively charged cellulose fiber and fillers resulting in increased fiber-to-fiber and fiber-to-filler bond strength important for papermaking. These starch also have applications in textiles and cosmetics, acting as thickening agents and emulsion stabilizers.
Crosslinking & Thermal Treatment of Starch
Crosslinking introduces new inter- and intra-molecular bonds on starch molecules in the granule, strengthening and stabilizing the granule. The benefit conferred to the starch is increased resistance to high or low temperatures and pH, maintaining the starch granule during processing since crosslinking decreases granule rupture and, consequently, loss of viscosity upon paste formation during cooking. These starches are commonly used in canned foods such as soups. Crosslinked compared to unmodified starches have decreased swelling capacity and solubility; however, resistance to retrogradation increases. Oxidation of starches converts hydroxyl groups to carbonyls (< 1.1%), which are either aldehydes or ketones, depending on their location, which impedes retrogradation. Oxidation of whole granules allows the granule to dissolve rather than swell and thicken, making them less susceptible to acidic environments. Succinylation with succinic acid anhydride increases paste viscosity and emulsion stability, lowers gelatinization temperature, and reduces glycemic response. It is used to stabilize beverages, mayonnaises, and battered meat.
Thermal treatments vary in temperature, presence of water, and annealing time, which alter starch structure differently. A key element of hydrothermal treatment is that the temperature must be above the glass transition but below the gelatinization temperature to maintain the starch granule size, shape and birefringence. Nonthermal modifications include shear (mechanical), pH, pressure ultrasonication and annealing (time)) which case structural changes to the granules, crystallinity and molecular structure, thereby decreasing digestibility and improving thermal and hydration stability and pasting characteristics. Both techniques tend to decrease digestibility and the basis for instant starches.
Starch Saccharification
Starch is suspended in water, heated to form a paste, and treated with amylase, which cleaves along the polysaccharides producing shorter dextrins by hydrolyzing the alpha (1 to 4) glycosidic bonds as the enzyme hydrolyzes glycosidic bonds, the hydrodynamic radius of the polysaccharide decreases, causing a decrease in solution viscosity. Recall that each polysaccharide in starch has a single reducing sugar, the first glucose in amylose or amylopectin; however, each time the polymer is hydrolyzed, a new reducing sugar at the end of the dextrin is created. Hence, colorimetric assays based on the Bendicts test are useful for monitoring the degree of hydrolysis. Additionally, the increase in reducing sugars (at the first glucose of each dextrin), a measure of the degree of hydrolysis, is calculated as a Dextrose Equivalent (DE). DE is the amount of reducing sugars present in a sugar product, expressed as a percentage on a dry basis relative to dextrose and ranges from 0, for starch, to 100 for glucose. Glucose syrups > 55 DE are ‘high conversion’; 35 < DE < 55 are ‘regular conversion’; and below 20 DE are maltodextrins. A maltodextrin with a DE of 4 has an average molecular mass of 3600 g mol-1; a DE of 10, the molecular mass decreases to 1800 g mol-1; and a DE of 20, the molecular mass decreases to 900 g mol-1.

To illustrate the decreasing phase transition temperature, the glass transition temperature (Tg) for DE 4 is -5oC, which decreases to -11oC and -16oC for DE 10 and 20, while DE100, glucose, is below -40oC. Maltodextrins, 3 < DE < 20, decrease in viscosity and increase in solubility as DE increases, but the polymers are still too long to be sensed as sweet. When the desired DE is achieved, the solution is spray-dried into a fine powder for maltodextrin. Maltodextrins are commonly used as bulking agents for pharmaceuticals and packets of sweeteners and as a thickener or filler to increase the volume of processed foods. DE > 20 are glucose syrups until DE 100, which is pure glucose, and commonly used high DE glucose syrups include DE 42 – 55 are confectioners syrup. Glucose DE 42 is a powder, ~40% as sweet as sucrose, but the variation in polymer length impeeds crystallization more than higher-DE glucose syrups and glucose. To obtain mono and disaccharides following alpha-amylase hydrolysis, pullulanase and isoamylase are added to cleave the alpha (1 to 6) glycosidic bonds. The resulting mixture of maltose and glucose can be separated and sold as either maltose or glucose or can be further treated with glucoamylase to obtain pure glucose. Throughout saccharification, the process is halted to obtain the desired mixture, and at any stage of hydrolysis, D-glucose keto isomerase converts the glucose syrup to high fructose corn syrup and is done to increase the sweetness. While many of these products reach the processed food market, significant amounts of glucose are fermented by bacteria and yeast to produce alcohol, amino acids and other compounds.