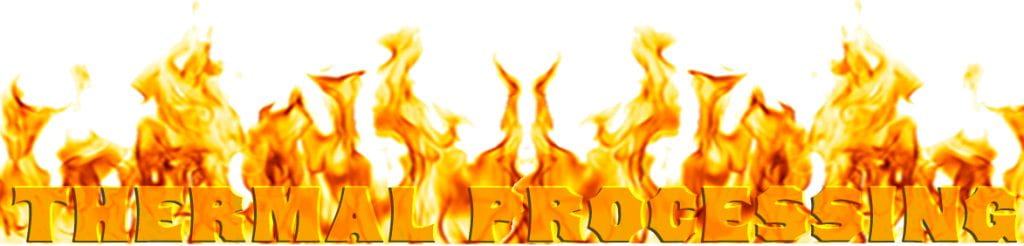
Blanching, Pasteurization and Commercial Sterilization
Thermal processes vary in lethality, and various time-temperature combinations are subdivided based on their intended use, where blanching is the mildest process and increases in lethality for pasteurization and commercial sterilization. Blanching is a mild heat treatment, typically done by immersing food (fruits and vegetables) in boiling water or atmospheric steam (100 oC) for a few minutes, not preserving food indefinitely. Once produce is washed, sorted and the size reduced (cutting, dicing, slicing), blanching releases entrapped air, drastically reducing the volume (frozen vs. fresh spinach) and inactivating enzymes before preservation processes such as drying and freezing are applied. Pasteurization inactivates vegetative pathogenic microorganisms that cause food-borne illness while spoilage and heat-resistant spore-forming bacteria remain active, and since it is a more intensive process than blanching, it also denatures endogenous enzymes. Pasteurization does not produce shelf-stable food; thus, they require additional hurdles such as refrigeration and fermentation to extend their best-before-expiry date. Commercial sterilization kills all viable microorganisms capable of growing in the food, and the severity of the process depends on the pH of the food and redox potential (e.g., aerobic vs. anaerobic vs. microaerophilic); microorganisms that cannot grow under the conditions of the food may survive the process and is why commercial sterilization is used in place of sterilization. Commercial sterilization can be done in a package (e.g., can or tetra pack) or outside the package coupled with aseptic packaging in a controlled sterile environment.
Microbial Inactivation

Microbial death or inactivation, irrespective of the technique applied, its power, or the microorganism, never reaches absolute zero because the death rate follows first-order reduction kinetics. The reduction is exponential for microbial death on an arithmetic scale, and the inactivation rate is constant, even if the population varies. In other words, it takes the same time to reduce the microbial population from 10,000 to 1,000 as it does to reduce the population from 10 to 1.
Due to the inactivation profile, semi-log plots are used to obtain a linear profile in which the slope measures the power of reduction, allowing for comparison between techniques and is time to produce one order-of-magnitude decrease (90%) in viable organisms, and is the decimal reduction time or D-value.
D-values, Z-values, and the 12D Process
The decimal reduction time or D-value is the time needed, at a given condition (e.g., temperature, pressure, electric current), to achieve one log or a 90% reduction of microorganisms. Thus, after an exposure time of 1 D, only 10% of the organisms remain; after 2 D, 1% remain viable, and after 3 D, the population declines to 0.1% of the original population. While semilogarithmic scales are used, population decline follows the natural logarithmic (ln(x)=loge(x) as opposed to 10x=log10 x), therefore to obtain the D-value from the slope, the thermal death constant, K, must be corrected by ln(10) or -2.303 since the logarithmic and not natural logarithmic is plotted on the Y-axis. More simply, the D-value can be obtained from the inverse of the slope, and the time is constant for each log reduction.
Each microorganism has a different thermal tolerance, where vegetative cells have the shortest D value and thermophilic spores have the longest D-value, which is also uniquely temperature dependent. While the goal of thermal processing is the reduction in the microbial population, nutrients are also consequently degraded with characteristic D-values. For the canned food industry, the processing time is based on a 12 log reduction (12D Process) of Bacillus stearothermophilus, used as an indicator organism because it is more heat resistant than Clostridium botulinum; therefore, if the Bacillus spores are deactivated, it is safe to assume that the botulinum spores are also not viable.

The z-value is the temperature increase required to decrease the D-value by a factor of ten or 1 log and is a measure of the resistance of an organism to differing temperatures. The z-value is obtained from the reciprocal of the slope from a semilogarithmic plot of D-values versus temperatures, as opposed to the population versus time plot required to calculate the D-value.Knowing the D-value at one temperature and the z-value allows the calculation of the equivalency of two thermal processes.

Therefore, if the microorganism has a D-value(@121oC) of 1 min, and a z-value of 10oC, every 10oC increase will reduce the time by a factor of 10; thus, the D-value(@131oC) = 0.1 min and at 141oC further decreases to 0.01 min, conversely, decreasing the temperature to 111oC would increase the time to reduce the population to 10 min from 1 min at 121oC. Each of these time-temperature combinations results in identical population reduction. While lethality may be identical other changes such as nutrient degradation, formation of off-flavors, and loss of aromatic volatiles may necessitate one process over another.
Using D and Z values to Preserve Nutrients
Processing time is based on the deactivation of microorganisms, so if the vegetative microbe slope is considered first, from the graph, the Dvalue(121oC) = 1 min and decreases to a Dvalue (126oC) = 0.1 min (z value 5oC). Therefore to achieve a 12D process, it would need holding at 121oC for 12 min but at 126oC, it would only be held for 72 seconds or 1.2 min, and both time-temperature combinations produce the same lethality or microbial inactivation.
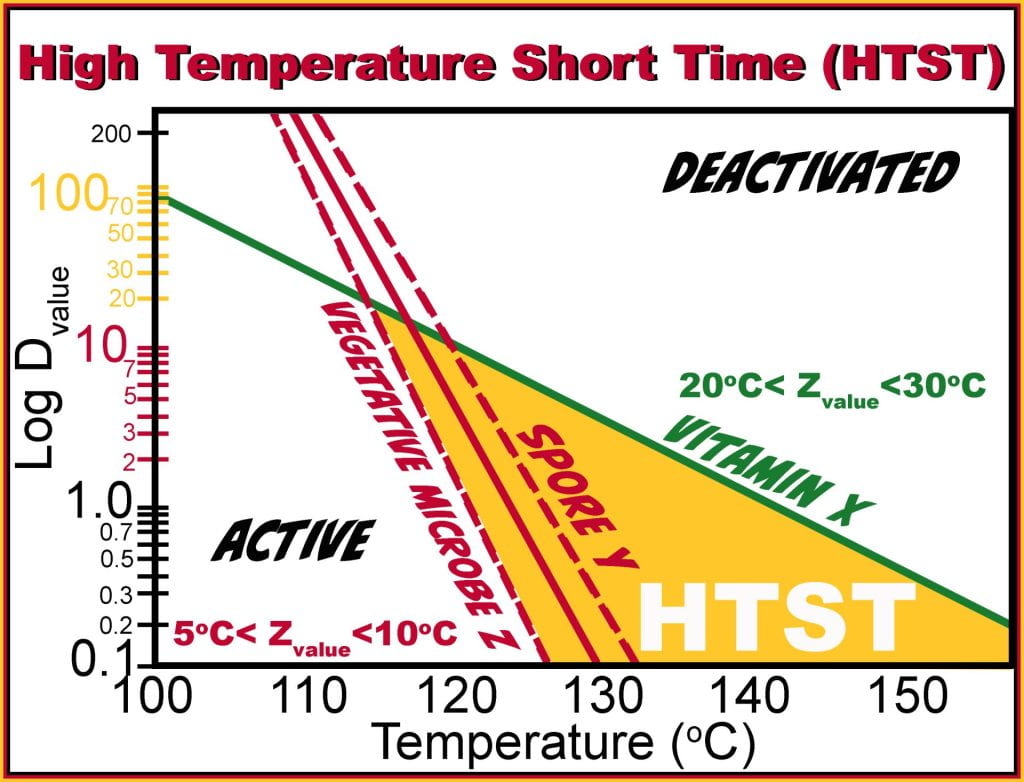
Dividing each holding time by the Dvalue for the vitamin at the same temperatures will give the number of log reductions of the nutrient under each condition. At 121oC, to inactivate the microbe, it takes 12 min, which is less the Dvalue of the nutrient (Dvalue (121oC) = 20 min); therefore, a (12min/20 min ) 0.6 log reduction in vitamin would be observed. At 126oC only (1.2min/7min), 0.17 log reductions of the vitamin occur, much lower than the 0.6 log reductions at 121oC; thus, higher temperatures and shorter times impart the same sterility while preserving nutrients.
Milk Pasteurization Considerations
Health Canada prohibits the sale of raw milk; however, unpasteurized milk cheese, made from raw or unpasteurized milk, is sold in Canada as the fermentation process eliminates harmful bacteria in raw milk. Milk is pooled at the farm level from multiple cows, several farms’ milk is collected in a single tanker truck, and then several trucks are pooled into the raw tank receiving before homogenization and pasteurization. Pasteurization is intended to eliminate pathogenic microorganisms while subsequently reducing spoilage microorganisms. Pasteurization eliminates gram-negative organisms from milk, while some thermophilic and mesophilic spoilage microorganisms survive. Low-temperature long-time (LTLT) processing occurs at 63oC for 30 min but has limited applications in dairy processing due to thermolytic modifications arising from Maillard reactions between lactose and proteins, resulting in the formation of brown pigments.
Long hold times denature milk proteins restricting the application in fluid milk processing. However, these changes, especially protein denaturation, may be desirable for small-scale ice cream, cheese and other dairy product manufacturing or producing soup broths where cooked flavors are desirable. LTLT is often done in small-scale batches using a heated vessel/kettle; the downfall to batch processing is the low surface area for heat transfer of the steam jacket kettle (Surface area = circumference x height), requiring a longer time to heat. The coldest spot in the kettle must reach 63 oC before the 30 min hold begins; the radius (meters, m) from the heat transfer surface to the coldest point is the characteristic dimension.

Juices, broths, fluid milk, and creams are processed continuously using high-temperature, short-time (HTST) pasteurization at 72°C for 15 seconds. HTST uses a plate heat exchanger, where the stainless steel plates are stacked together, and unpasteurized fluid travels between the plates in shallow channels. Channels are oriented to maximize turbulent flow, ensuring the constant exchange of molecules at the hot steel surface. The gaps (10-2, cm) between stacked plates alternate between raw product and steam, allowing heat transfer from both sides of the gap, creating a large surface area for heat exchange and a small characteristic dimension (half of the gap width (10-3, mm)). HTST allows continuous operation, a large surface area for heat exchange, and a small characteristic dimension to the coldest point. This design strategy allows for rapid heating and cooling, which preserves the nutritional and sensory quality of the product. HTST-processed milk and milk products have extended shelf life under refrigerated conditions but are not commercially sterile, as pasteurization eliminates pathogens and reduces the spoilage of microorganisms. Once an HTST operates, a fraction of energy is recycled by initially passing gaps, one with pasteurized milk and the other with cold raw milk, reducing the steam used in operation.
Ultra-high-temperature (UHT) processes milk at 140°C for 4 sec and is commercially sterilized rather than pasteurized allowing milk or other fluid, such as juice, to be stored for months without refrigeration. Commercial sterilization eliminates pathogenic and spoilage microorganisms capable of growing in sealed food containers. UHT processing atomizes fluid milk into small droplets as it enters the superheated pressurized heat exchanger. The atomized droplets are in direct contact with steam, drastically increasing the surface area for heat exchange while decreasing the characteristic dimension that heat must travel to the center of the droplet (10-6 m, µm), resulting in near-instantaneous heating (and cooling). UHT processing does not have a conductive barrier between the droplet and heated chamber; therefore, steam and product are in direct contact with each other, and steam condenses, increasing the volume of the milk. After the hold time, the hot pasteurized product enters a cooled chamber under a vacuum, causing evaporative cooling and eliminating the added water from the condensed steam. The commercially sterile product is cooled instantly in a vacuum chamber and must be aseptically packaged in a pre-sterilized, airtight container.