The Gut-Brain Axis
The digestive system comprises the gastrointestinal tract or alimentary canal, which contains the oral cavity, esophagus, stomach, small and large intestines, the rectum, and periphery organs, including salivary glands, liver, pancreas and gallbladder, which communicates via the gut-brain axis involving neural, endocrine and immune signaling pathways. In the oral cavity, digestion is initiated through mastication using the teeth, tongue and palate to chew the food while the submandibular, the largest of the salivary glands, predominately secretes saliva when unstimulated (fasted); upon food consumption or stimulation, the parotid salivary gland dominates secreting saliva, and the pair of sublingual glands, are the smallest and only produce 5% of the total saliva. Also, part of the oral cavity, the uvula, prevents food and water from entering the nasal cavity during swallowing. Swallowing passes the bolus through the pharynx past the vocal cords to the esophagus, which aids in bolus transit via weak peristaltic contractions. Once swallowed, the bolus resides in the stomach and is exposed to gastric secretions and strong peristaltic contractions, which further mix the bolus with water, HCl and enzymes, forming the chyme, which is an aqueous, acidic dispersion that must transit to the small intestine through the pyloric sphincter restricting particle size to less than 2 mm. The small intestine comprises the duodenum, jejunum, and ileum, connected to the pancreas and gallbladder, which secrete enzymes and bile (produced in the liver) via the pancreatic and bile ducts. The large and small intestines connect through the cecum, a small pouch that acts as a reservoir before transiting through the transverse, ascending, descending and sigmoid colons and the cecum and rectum. The remaining anatomy of the digestive system is the appendix and anus.

The gut-brain axis involves neural, endocrine and immune signaling pathways. The nervous system contains a connected network of cells, tissues and organs subdivided into the autonomic, parasympathetic, and enteric systems. The autonomic nervous system is connected to organs, muscles and glands controlling the heart and respiratory rates, urination and the fight-or-flight response. Unlike the autonomic fight-or-flight response, the parasympathetic nervous system relies on the vagus nerve to control the body during rest, acting on glands in the oral cavity to produce mucin, on the intestines to increase blood flow and peristalsis (muscle contractions), on the pancreas to release insulin, and on relaxing muscles involved in urination and defecation.
The enteric nervous system includes efferent, afferent, and interneurons embedded in the lining of the gastrointestinal system from the esophagus to the anus coordinating reflexes and operating independently of the brain and spinal cord. Unlike the nervous system, the endocrine system is a network of disconnected glands and organs that communicate and coordinate metabolism, energy level, mood and response to stress and injury via circulating hormones. These hormones act on specific centers in the brain, such as neuropeptide Y (NPY), acting on the hypothalamus, the control center for hunger and satiety. Hormones involved in the hunger response include NPY, agouti-related peptide (AgRP), and ghrelin, while hormones aligned with the satiety response include pro-opiomelanocortin (POMC) and cocaine- and amphetamine-regulated transcript (CART) which act on the hypothalamus and peripheral hormones cholecystokinin (CCK), polypeptide YY (PYY), pancreatic polypeptide PP, glucagon-like peptide-1 (GLP-1), insulin and leptin (Austin and Marks, 2009). Cytokines, produced by T-cells and macrophages, are small protein fragments that mediate homeostasis and immune control as part of the innate immune system acting via an intricate interplay with mutually dependent positive and negative feedback mechanisms detectable in the gastrointestinal mucosa. Cytokines play an important role in gastrointestinal motility and inflammation.
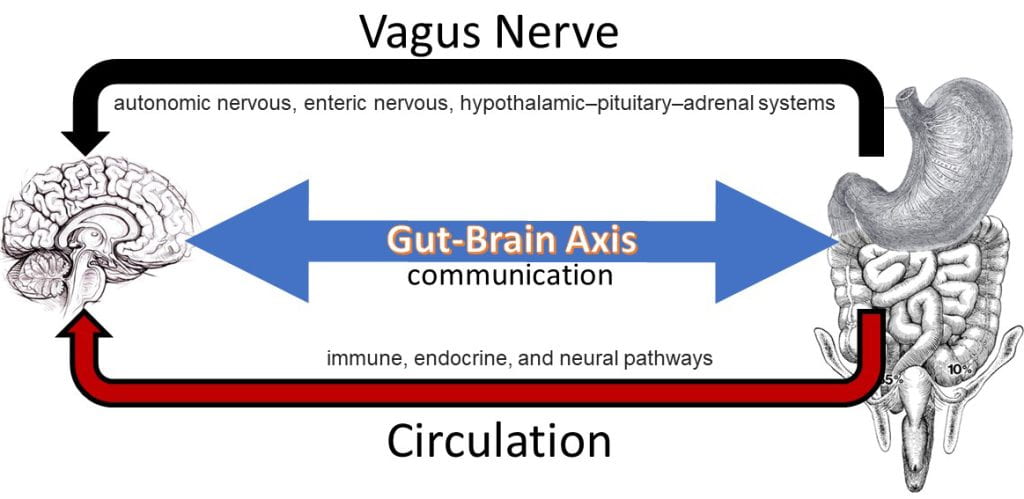
Hunger, Appetite, Satiation and Satiety
Long- and short-term antagonistic processes regulate food intake. Appetite, the long-term desire to satisfy the bodily need for glucose and energy, is extremely complex and must account for the cultural, physiological, psychological, sensory, and social influences on the selection and intake of food, all of which are influenced by the food environment. Appetite is often tied to our psychological drive to eat. Hunger, or our biological drive to eat, is triggered when low blood glucose is detected in the brain, which triggers the release of NPY. NPY regulates food intake, alters mood and energy homeostasis, and is sensed by the stomach, increasing gastric motility (antral wave contractions or peristalsis). Food consumption is initiated (short-term) by hunger following the production of NPY and regulated (long-term) by appetite. Hunger often concurs with the cephalic response, which is triggered by the thought, smell, or sight of food, communicating to gastrointestinal tract through the vagus nerve to prepare to receive food. The cephalic response triggers endocrine cells in the stomach to release gastrin starting a cascade that acts on chief and parietal cells to release the inactive zymogen (pepsinogen) and HCl, respectively. Upon mixing the gastric fluid is acidified and protease enzymes are activated (pepsin) to begin digestion. As part of the cephalic response, salivary secretions increase; remarkably, prior to Ivan Pavlov’s experimentally proven theory of conditioned reflexes using surgical fistulas to study the salivary glands of dogs’, psychic salivary secretion was the school of thought. Pavlov’s 1904 Nobel Prize in medicine for developing the surgical use of fistulas to study functions of various organs showed that the nervous system plays the dominant part in regulating the digestive process, marking the onset of our modern understanding of the physiology of digestion while discovering that reflex mechanisms were not of psychic activity but instead conditioned reflexes.

Upon food consumption, the gastric cavity expands, distension initiates the vagovagal reflex. The vagovagal reflex communicates fullness via mechanosensitive and other vagal afferents, which are the neuronal component of the gut–brain axis allowing information to flow from the GI and viscera to the central nervous system. Distension triggers the release of acetylcholine causing the relaxation of muscles along the gastrointestinal tract allowing for further distension and additional acid production in the stomach. The reflex is the first cue to initiate satiation, the short-term drive to stop eating, or your perception of fullness. The meal slowly and incrementally starts to transit to the duodenum where the chyme is neutralized via secretion of sodium bicarbonate and exposed to an array of digestive enzymes. As macromolecules (triglycerides, digestible carbohydrates, and proteins) are assimilated they are sensed by taste cells that line the GI triggering the ileal break, another satiation cue. Post prandial nutrient sensing in the small intestine triggers the ileal break, causing the release of cholecystokinin (CCK) and pancreatic polypeptide (PP) from the intestinal lining initiating negative feedback mechanisms that influence the function of the proximal gastrointestinal tract. CCK, in addition to suppressing appetite, also is sensed by gallbladder, causing the contraction and release of bile, and on the pancreas stimulating the release of digestive enzymes. Satiety is the long-term perception of fullness following a meal until the return of hunger. Once the meal completely transits from the stomach to the small intestine and the stomach is empty, ghrelin, the hunger hormone is released initiation food seeking behavior and the return of hunger.

Evolutionary Pressure to Over Consume Calories
An evolutionary framework for why traditional hunter-gather diets evolved to over consume sweet and high-fat palatable foods preferentially arose due to their limited supply, prolonged time spent foraging and hunting, and the capacity to find these foods pleasurable motivated ancestral humans to engage in food-seeking behaviors (Appelhans, 2009). Before the onset of agriculture and most of human evolution, traditional hunter-gather diets evolved to preferentially over consume sweet and high-fat palatable foods as early hominids were under constant risk of famine and satiety signals were overridden, facilitating overconsumption of calories to be stored as fat acting as an effective buffer against starvation, giving rise to hedonic feeding (i.e., our drive to eat to attain pleasure) (Berthoud, 2007).
Feeding behavior is controlled by three brain regions: the prefrontal cortex, mesolimbic dopamine system, and hypothalamus. The prefrontal cortex controls decision-making and processes social cues such as food environment, price, and availability. The mesolimbic system underlies the motivational drive to consume palatable food (i.e., dopamine increases and overrides homeostatic controls), and their consumption can take over the endogenous reward system to promote hedonic feeding; although controversial, herein lies the concept of food addiction (Fletcher & Kenny, 2018). Molecular and neural mechanisms that regulate body weight homeostasis controlling food intake require signals about peripheral energy levels (Zigman & Elmquist, 2003). Peripheral signaling uses a series of circulating hormones to communicate energy status via the gut-brain axis through the regulation of mesolimbic dopamine signaling (Lutter & Nestler, 2009). The hypothalamus is acted up by regulatory neuropeptides leptin, cholecystokinin (CCK), ghrelin, orexin, insulin, neuropeptide Y (NPY) and nutrient sensing(i.e., glucose, amino acids, fatty acids).
Consumption of either highly palatable foods or drugs of abuse converges within the limbic system to mediate motivated behaviors (Nestler, 2005). In the case of highly palatable foods, their visual presentation is sufficient to induce a potent release of dopamine from nerve terminals originating in the ventral tegmental area (VTA) onto neurons in the nucleus accumbens (NAcc) (Nestler, 2001). Activation of VTA dopamine neurons may also occur on exposure to the peptide neurotransmitter orexin, expressed by the lateral hypothalamic (LH) neurons that supply much of the brain, including the VTA, with neurons (Harris et al., 2005). Dopaminergic transmission either directly stimulates the dopaminergic neurons or indirectly through inhibition of GABAergic interneurons in the VTA (Harris et al., 2005). Remarkably, a hyperactive reward system significantly increases activation of the medial and lateral orbitofrontal cortex, amygdala, NAcc, medial prefrontal cortex, insula, anterior cingulate cortex, ventral pallidum, caudate, putamen, and hippocampus increasing motivation potency of high-calorie foods in obese compared to normal weight women (Stoeckel et al., 2008). Chronic consumption of highly palatable foods alters brain function (in a similar fashion as drugs of abuse), specifically within the mesolimbic dopamine reward pathway (Lutter & Nestler, 2009). The long-term consequences on limbic function and motivation behaviors may yield important insights into compulsive eating (Monteiro et al., 2010).
A neural circuit model for obesity suggests overeating arises due to the imbalance between circuits that motivate behavior, via reward and conditioning, with circuits that control and inhibit pre-potent responses (Volkow et al., 2013;). Palatable foods can upset the balance of main circuits (e.g., reward–saliency; motivation–drive; learning–conditioning; and inhibitory control–emotional regulation–executive function), resulting in an enhanced reinforced value of food that overrides self-control mechanisms and leads to addiction (Volkow et al., 2011). As populations shift into food-rich environments, individuals are constantly exposed to ‘cue-triggered urges,’ potentially contributing incrementally to the long-term overconsumption of calories (Berridge et al., 2010). Intense sweetness, initiated by sucrose or saccharine, commonly found in sweetened beverages and UPFs, stimulate T1R2 and T1R3 G-protein-coupled subunit receptors on the tongue, generating a supranormal reward signal in the brain, surpassing that of even cocaine (Lenoir et al., 2007)!
Works Cited
Austin, J., & Marks, D. (2009) Hormonal Regulators of Appetite. International Journal of Pediatric Endocrinology. 2009,141753.Appelhans, B. M. (2009). Neurobehavioral Inhibition of Reward-driven Feeding: Implications for Dieting and Obesity. Obesity, 17, 640-647.
Berridge, K. C., Ho, C.-Y., Richard, J. M., & DiFeliceantonio, A. G. (2010). The tempted brain eats: Pleasure and desire circuits in obesity and eating disorders. Brain Research, 1350, 43-64.
Berthoud, H.-R. (2007). Interactions between the “cognitive” and “metabolic” brain in the control of food intake. Physiology & Behavior, 91, 486-498.
Fletcher, P. C., & Kenny, P. J. (2018). Food addiction: a valid concept? Neuropsychopharmacology, 43, 2506-2513.
Harris, G. C., Wimmer, M., & Aston-Jones, G. (2005). A role for lateral hypothalamic orexin neurons in reward seeking. Nature, 437, 556.
Lutter, M., & Nestler, E. J. (2009). Homeostatic and hedonic signals interact in the regulation of food intake. The Journal of Nutrition, 139, 629-632.
Monteiro, C. A., Levy, R. B., Claro, R. M., de Castro, I. R. R., & Cannon, G. (2010b). Increasing consumption of ultra-processed foods and likely impact on human health: evidence from Brazil. Public Health Nutrition, 14, 5-13.
Nestler, E. J. (2005). Is there a common molecular pathway for addiction? Nature Neuroscience, 8, 1445.
Stoeckel, L. E., Weller, R. E., Cook, E. W., Twieg, D. B., Knowlton, R. C., & Cox, J. E. (2008). Widespread reward-system activation in obese women in response to pictures of high-calorie foods. NeuroImage, 41, 636-647.
Volkow, N. D., Wang, G.-J., & Baler, R. D. (2011). Reward, dopamine and the control of food intake: implications for obesity. Trends in Cognitive Sciences, 15, 37-46.
Volkow, N. D., Wang, G. J., Tomasi, D., & Baler, R. D. (2013). Obesity and addiction: neurobiological overlaps. Obesity Reviews, 14, 2-18.
Zigman, J. M., & Elmquist, J. K. (2003). Minireview: From Anorexia to Obesity—The Yin and Yang of Body Weight Control. Endocrinology, 144, 3749-3756.