Lipid Sources
Lipids are essential in the body, providing energy, acting as a structural component of cell membranes, as messenger molecules involved in cell signaling, and delivering fat-soluble vitamins (Vit A, D, E, and K). Dietary lipids are high in whole foods such as nuts and seeds, meat and fatty fish, eggs, dairy products, fruit such as avocado, palm and olives, and whole grains. In whole foods, lipids are localized in cellular structures; for example, in plant cells (e.g., seeds, fruit and leaves), lipids are stored in small oil bodies, typically spherical or ellipsoidal organelles surrounded by a membrane of phospholipids and proteins. Lipids from animal sources are stored as adipose tissue and found in the connective tissue surrounding muscle fibers, affecting flavor, tenderness, juiciness and bioavailability. In meat, marbling is the distribution of fat surrounding muscle fibers characterized by small white flecks or streaks of fat interspersed within muscle fibers.
Dairy products, which are oil-in-water emulsions, are surrounded by the milk fat globular membrane, comprised of surface-active molecules ranging from low molecular weight phospholipids to macromolecules such as protein. Fats and oils are refined from numerous sources to formulate ultra-processed foods. Various liquid oils are extracted and refined from corn, canola, soy, olive and sunflower oil. However, solid fat sources are much more limited, including lard, animal tallow, butter, ghee and fractioned milk fat from dairy, egg yolk, cocoa butter, and fractioned palm and coconut oils. As of 2023, there is a dietary fat shortage, as avian flu has led to a major shortage of eggs; palm oil exports are being tightly regulated to combat deforestation; trans fats are banned in Canada, while cocoa butter and animal fats costs continue to rise at rates greater than inflation. New sources of fats and oils that can be refined and modified into functional ingredients are rapidly emerging from single-cell oils, including bacterial, algal and yeast, to insects such as cockroaches and soldier-fly larvae.

Lipid Categories

Lipids are organic molecules insoluble in water but soluble in organic solvents, including nonglyceride lipids (e.g., waxes, sphingolipids, and steroids), fatty acids and their glycerides (e.g., triglycerides and phospholipids) and complex lipids (lipoproteins). While many of these molecules have relevant biological roles and are considered phytochemicals or bioactives, only fats and oils and their fatty acids and triglycerides will be further elaborated.
Lipids interact via weak Van der Waals interactions that arise from fluctuations in the electron density of the atoms or molecules, which can cause temporary dipoles. The temporary dipoles induce similar dipoles in neighboring atoms or molecules, resulting in a net attraction between long hydrocarbon chains. Van der Waals interactions are incompatible and do not interact with permanent dipoles found in water and other polar molecules. Mixing lipids and water give rise to hydrophobic intermolecular forces generated from the tendency of nonpolar molecules, or parts of molecules, to avoid contact with water molecules. Hydrophobic force is the entropy-driven tendency of water molecules to minimize contact with nonpolar substances, and in aqueous solutions, hydrophobic molecules tend to aggregate together, forming clusters or micelles, in order to reduce their contact with water.
Saturated Fatty Acids
Dietary fatty acids, H(CH2)nCOOH, contain an even number of carbon atoms because they are synthesized using acetyl groups, a two-carbon molecule attached to co-enzyme A (Acetyl-CoA). During fatty acid synthesis, multiple acetyl groups from acetyl-CoA molecules are added sequentially to form longer fatty acid chains that always have an even number of carbon atoms. Each CH2 group contributes a weak Van der Waals attraction; its solid-liquid melting temperature increases as the chain length increases. Short-chain fatty acids (SCFA), acetic (C2), propanoic (C3), and butyric (C4) acids are volatile fatty acids that can be oxidized to produce energy by the cells of the epithelial lining of the gastrointestinal tract. SCFAs are high in cow milk; when butter goes rancid, butyric acid is hydrolyzed from glycerol, giving rise to an unpleasant odor and acrid taste and is the characteristic smell of parmesan cheese. Fatty acids, including butyric acids and acetic and propanoic acids, are formed in the colon by bacterial fermentation of carbohydrates such as dietary fiber, which are shown to suppress colorectal cancer. Medium chain fatty acids (MCFAs) include caproic (C6), caprylic (C8), capric (C10), and lauric (C12) acids and are sourced from palm kernel and coconut oils.

MCFAs are also found in milk fat from cows, sheep, and goats at approximately 10-20% of the total fatty acids. MCFAs melt at higher temperatures than SCFAs and are either oily liquids (caproic acid m.p. -3.4 oC and caprylic acid m.p. 16.7 oC) or solid fat (capric acid m.p. 31.6 oC and lauric acid m.p. 43.2 oC) at room temperature. Lipase (lingual, gastric and pancreatic) preferentially hydrolyze fatty acids located at the sn1 and sn3 ester bonds of the triglycerides, which have higher activity for short- and medium-chain fatty acids (MCFA) than for long-chain fatty acids (LCFA).
MCFA absorbed along the small intestinal cells can be transferred directly into the portal vein and the liver, where they undergo hepatic metabolism or mitochondrial β-oxidation. β-oxidation cleaves off an acyl (C2) using CoA and yields 5 molecules of ATP during oxidative phosphorylation. Conversely, LCFA and 2-monoglycerides are re-esterified into triglycerides and incorporated into chylomicrons, which enter lymphatic circulation and are either oxidized for energy or stored in adipose tissue. The difference between how MCFA and LCFA are absorbed has been proposed as useful for subjects with impaired fat digestion and absorption. LCFAs are solid at room temperature and range in length from 14 carbons (myristic acid) to 20 carbons (arachidic acid) and above C22 (behenic acid); they are classified as very long-chain fatty acids (VLCFA). Two LCFAs, palmitic (C16) and stearic acids (C18), can be desaturated via the enzyme stearoyl-CoA desaturase-1 or Δ9 desaturase to monounsaturated lipids in the endoplasmic reticulum when they are not oxidized or stored in adipose tissue. LCFAs are solid at room temperature and range in length from 14 carbons (myristic acid) to 20 carbons (arachidic acid) and above C22 (behenic acid); they are classified as very long-chain fatty acids (VLCFA). Two LCFAs, palmitic (C16) and stearic acids (C18), can be desaturated via the enzyme stearoyl-CoA desaturase-1 or Δ9 desaturase to monounsaturated lipids in the endoplasmic reticulum when they are not oxidized or stored in adipose tissue.
Unsaturated Fatty Acids
Unsaturated lipids, considered essential macronutrients with desirable outcomes for health, have cis-configuration, where the hydrogens are on the same side of the double bond, creating a kink in an otherwise straight aliphatic chain.There are currently two commonly applied names to identify the double bond(s) position on the aliphatic chain of carboxylic acids.
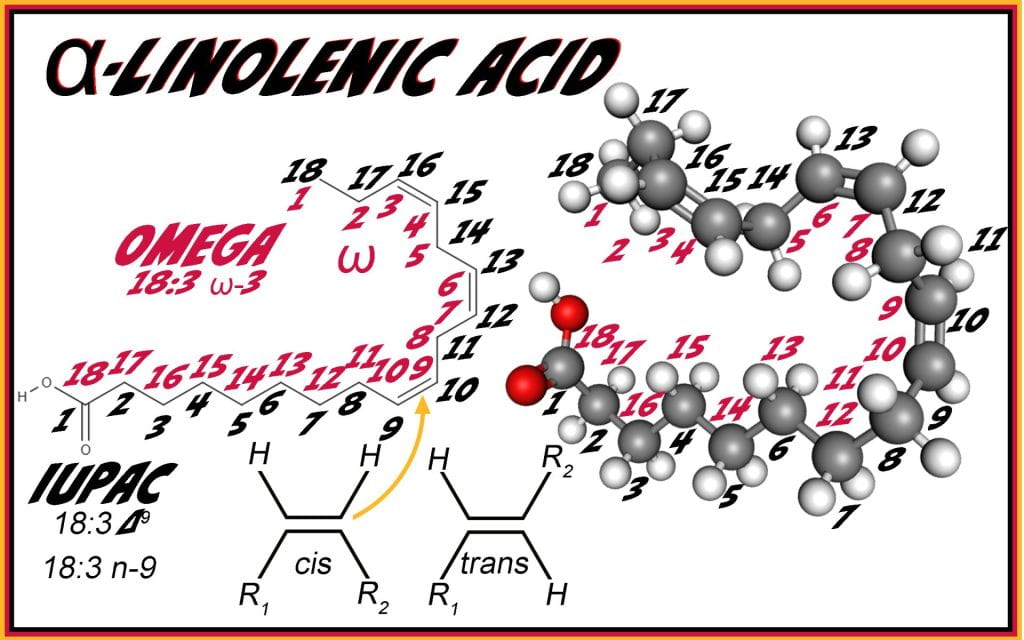
IUPAC naming starts at the carboxylic acid, while omega starts numbering from the methyl end of the fatty acid. In the case of α-linolenic acid, IUPAC convention 18:3 n-9 identifies the molecule as having 18 carbons, 3 double bonds where the conjugation (-double-single-double-single-) starts at carbon 9 from the carboxylic acid end of the molecule and has the last double bond three carbons away from the methyl end of the molecule and an omega-3.
Monounsaturated fatty acids have one double bond. As mentioned briefly above, palmitic and stearic acids can be desaturated in the body using stearoyl-CoA desaturase-1 to form either palmitoleic or oleic acids. Polyunsaturated fatty acid synthesis requires dietary sources of linoleic (18:2 n-6) and α-linolenic (18:3 n-9), which can be further desaturated and elongated by two carbon units. Δ6 desaturase adds a double bond to 18:2 n-6 producing 18:3 n-6 (γ-linolenic acid); next elongase adds an two carbons (20:3 n-6) followed by Δ5 desaturase (20:4 n-6), elongase (22:4 n-6), and Δ4 desaturase (22:5 n-6). The same enzymes act on α-linolenic acid, eventually generating EPA (20:5n-3) and DHA (22:6 n-3).
The body does not produce trans unsaturated fatty acids, and their consumption through dietary sources is considered undesirable. The primary source of trans fats until recently was partially hydrogenated vegetable oils, high in elaidic acid, which are now banned in Canada. The majority of trans fat consumption now is found in milk products as vaccenic acids and conjugated linolenic acid (CLA), which has a trans and one cis configuration double bond.

Effect of Fatty Acid Structure on Melting Profile
The melting point of fatty acids depends on the carbon chain length and the degree and type of unsaturation. Increasing the fatty acid carbon chain length increases the melting points (C4 m.p. -3.4 oC, C12 m.p. 43.2 oC, and C18 m.p. 69.3 oC) because the Van der Waals intermolecular forces are proportional to the carbon chain length. Saturated fatty acids have higher melting points than unsaturated fatty acids as they arrange more closely in the crystal lattice, generating greater van der Waals attraction between adjacent molecules. The presence of cis-configuration double bonds in unsaturated fatty acids creates kinks in their hydrocarbon chain, which impedes the ability of the molecule to pack together, thereby decreasing the melting point.

As the number of double bonds increases, the melting temperature of the fatty acid decreases; for example, stearic acid, 18:0, melts at 69.3 oC; oleic acid, 18:1, at 13.4 oC, linoleic acid, 18:2, at -5.0 oC, and α-linolenic, 18:3, melts at ‑11.3oC. Compared to the cis configuration, trans-unsaturated fatty acids melt at higher temperatures; for example, oleic acid contains the cis configuration and melts at 13.4 oC, while the trans isomer, eladic acid, melts at 52 oC, which is closer to the saturated fatty acids (18:0 melts at 69.3 oC). While the fatty acid composition of a lipid is important in determining the melting point of the fat, the position and distribution of fatty acids on glycerol must also be considered, as this alters the melting profile of the fat.
Triglycerides
Dietary fats and oils contain triglycerides (aka triacylglycerides) with three fatty acids esterified onto each alcohol group of glycerol. Esterification is a condensation reaction between the hydroxyl (-OH) groups of the glycerol and the carboxylic acid (-COOH) of fatty acids forming an ester bond (R−C(=O)−O−R) and liberating a water molecule. The resultant triglyceride consists of three fatty acids attached to each of the three carbon atoms of the glycerol backbone. The fatty acid position on glycerol is defined using the stereospecific numbering (sn) system as sn1, sn2 and sn3 from a Fischer projection.
Sn2 is the central fatty acid; if the molecule is symmetric, meaning the same type of fatty acids is located at sn1 and sn3, then discerning their position is irrelevant; however, for asymmetric triglyceride (TAGs), the larger fatty acid is assigned to the sn1 position. Both sn1 and sn3 are hydrolyzed preferentially by lipase, which liberates two free fatty acids and one 2-monoglyceride. Furthermore, delineating sn-1 and sn-3 is important as it alters the physical and chemical properties of TAGs, such as melting point, crystal polymorphism and reactivity.


The distribution of fatty acids available to be esterified differs based on the strain and species. For example, canola oil is approximately 60% 18:1, 20% 18:2, 10% 18:3, 5% 16:0 and 5% 18:0. Palm oil, on the other hand, has 50% 16:0, 40% 18:1, 5% 18:0, and 5% 14:0. Finally cocoa butter contains three fatty acids in almost equal proportions ~40 % oleic, 30% stearic and 30% palmitic acids and contains small amounts (<2%) of 18:2. The biosynthetic pathway of edible plants and animals, which assembles TAGs from fatty acids and glycerol, does not evenly nor randomly place fatty acids evenly at each sn position; instead, a restricted random distribution is observed for most fats and oils. Restricted random distributions have a degree of preference (bias) towards placing certain fatty acids at each sn position, but the distribution is still considered random. The best illustration of this distribution is cocoa butter, which clearly prefers unsaturated fatty acids, 18:1 and 18:2, to be placed at sn2.
The regioselectivity and amounts of each fatty acid in cocoa butter result in almost every TAG molecule containing an unsaturated and two saturated fatty acids. The two saturated fatty acids, 16:0 (m.p. 62.9oC) and 18:0 (m.p. 69.3oC), have similar melting points, and when combined on a TAG with oleic acid melt at 32 oC, or body temperature and why chocolate melts in your mouth!